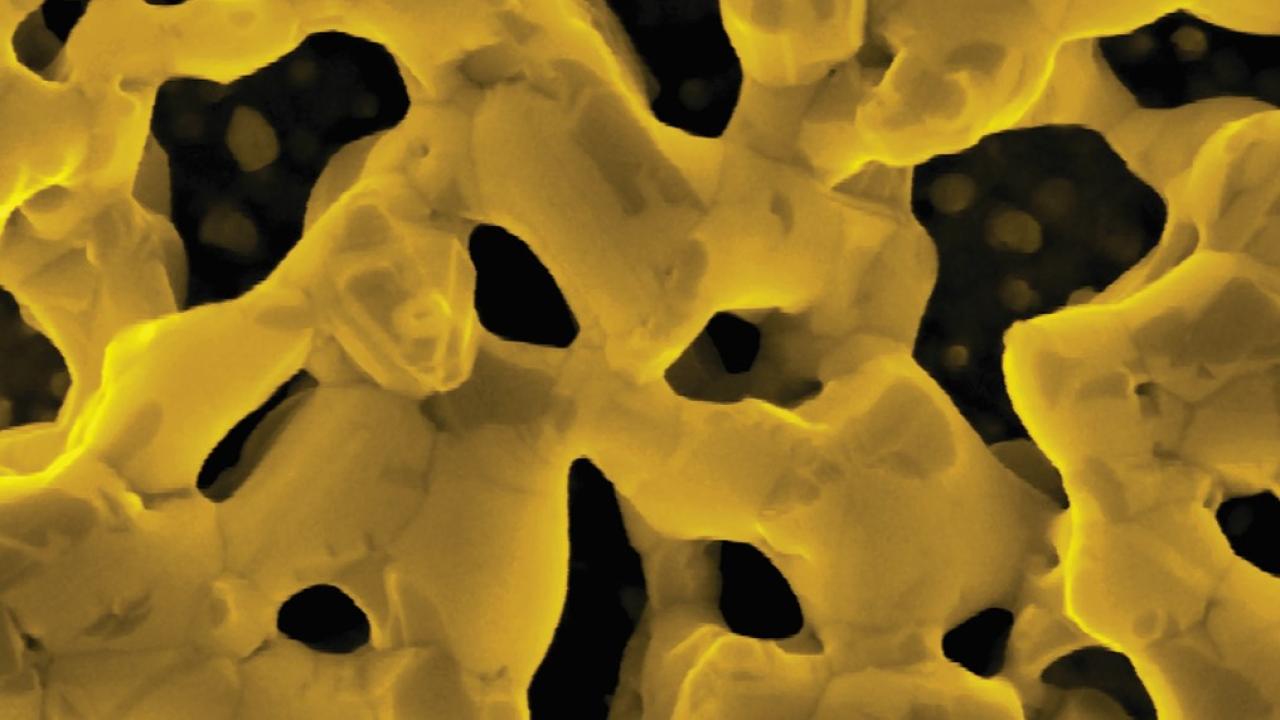
UC Davis engineers receive NSF funding to study properties of nanoporous gold
A new NSF-funded project at UC Davis aims to give researchers “recipes” for consistent and optimized structures of nanoporous gold—a material with potential applications in a variety of fields. Electrical and computer engineering associate professor Erkin Şeker and materials science and engineering assistant professor Jeremy Mason will use experimental and computational methods to study how thermal, mechanical and electrical forces impact the structure to find the strings researchers can pull to create the structures they need.
“We’re going to see what kinds of structures we can cook up in this nanoporous gold,” said Mason. “If we understand these three mechanisms and we understand how they change the structure of the sample, we could give you a recipe to construct structures for a specific application.”
Nanoporous gold contains microscopic interconnected tunnels that run throughout the material, making it look like a sponge. Its huge surface area gives it potential to be a catalyst or to store pharmaceuticals by giving molecules lots of places to bind, and its pitted surface can be tailored to allow specific types of cells to attach, raising possibilities in biomedical engineering as well.
However, researchers currently don’t have much control over the structure, so figuring out how to manipulate it for different applications or produce a given structure more consistently is an obstacle that Şeker and Mason hope to overcome.
To do this, Şeker will design and perform experiments to see how the gold responds to stretching in different directions (mechanical stress), heating one side more than the other (temperature gradient), and passing an electrical current through (Joule heating) both independently and simultaneously. Meanwhile, Mason will conduct molecular dynamics simulations of each experiment to understand how the external forces impact the energies and movements of individual atoms in the system.
“You can see the effect in experiments, but if you don’t have the numbers [from the models], you can’t predict what will happen under different circumstances,” Mason explained. “Our goal is to use analytical models to extrapolate from what’s happening to one individual atom to an equation which will hopefully describe, explain and encapsulate Dr. Şeker’s results.”
The project is a true collaboration, as Mason will help Şeker understand what’s happening at the molecular level in his experiments and make informed choices about which experiments to run, while Şeker will give Mason a “reality check” for his models and verify their accuracy.
“Erkin [Şeker] is bringing ideas and methodologies and training that I don’t have to this project, and similarly for me, and that enables us to do things that couldn’t have been done otherwise,” he said.
Mason and Şeker are also excited to involve their undergraduate students in the process and give them hands-on experience on both the experimental and computational sides. Both researchers plan to recruit from their undergraduate classes this fall.
“This is a fantastic opportunity for undergraduates,” said Mason. “One of the best ways to disseminate knowledge is to bring people in to see it firsthand, so I want undergraduates to see how this works and be involved in it as early as possible.”
Funding for the three-year, $480,000 project titled, “Experimental and Computational Study of Pore Morphology Evolution Mechanisms in Nanoporous Metal Thin Films Under Thermal/Electrical/Mechanical Stress Fields” began July 1.